Endurance Training and Body Transformation: Metabolic, Muscular, and Cardiovascular Changes Explained
Discover how endurance training transforms your body at the cellular level. Explore key metabolic, muscular, and cardiovascular adaptations backed by research—and learn how to optimize your performance through science-based strategies.
DR T S DIDWAL MD
4/9/202510 min read
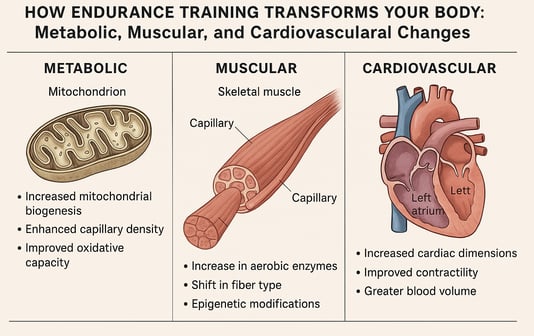
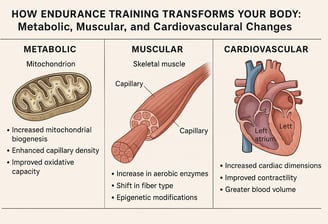
Endurance Training: A Comprehensive Look at Physiological Adaptations, Protein Needs, and Metabolic Changes
Endurance training represents one of the fundamental pillars of athletic performance. Whether you're a marathon runner, cyclist, swimmer, or cross-country skier, understanding the science behind endurance can help optimize your training and performance. This article explores the latest research on physiological adaptations, protein requirements, and metabolic changes that occur with endurance training.
Endurance Training transforms your body in powerful ways—enhancing your cardiovascular function, muscle metabolism, and even your genetic expression. Whether you're training for a marathon or looking to improve general fitness, understanding the science behind endurance can sharpen your strategy and boost results.
Regular endurance exercise leads to key physiological adaptations, such as increased mitochondrial biogenesis, enhanced capillary density, and improved VO₂ max, all of which enhance aerobic energy production and delay fatigue. Training type matters: Sprint Interval Training (SIT) and High-Intensity Interval Training (HIIT) are shown to increase mitochondrial respiration more efficiently than traditional long-duration workouts.
Don’t overlook protein needs—especially during carbohydrate-restricted training. Endurance athletes should aim for ~1.8–2.0 g/kg/day to support muscle recovery and training adaptation. While adding protein during workouts doesn’t boost performance much, post-exercise intake is key.
Endurance training also rewires your metabolic profile, increasing fat utilization and changing levels of key metabolites like acylcarnitines and bile acids. On a deeper level, epigenetic modifications such as DNA methylation and histone acetylation play a pivotal role in long-term adaptation.
Bottom line? To thrive in endurance sports, optimize training intensity, protein intake, and personalized nutrition—and let the science fuel your success.
Understanding Endurance Exercise
Endurance exercise is broadly defined as any physical activity that increases your heart rate above 50% of your maximum for prolonged periods. The fundamental requirement for any endurance sport is the ability to sustain a submaximal work rate over time.
Endurance can be categorized into two main types:
General endurance: The ability of the whole body to tolerate prolonged efforts across various disciplines
Specific endurance: The ability to resist fatigue in sport-specific conditions
Success in endurance sports depends on several physiological factors, including:
Efficiency of energy systems
Aerobic capacity/VO2 max
Lactate threshold
Muscle strength and power
Muscular endurance
Physiological Adaptations to Endurance Training
Skeletal Muscle Adaptations
Endurance training triggers significant adaptations in skeletal muscle that enhance performance and delay fatigue:
Increased mitochondrial biogenesis: More mitochondria mean more efficient energy production
Enhanced capillary density: Better oxygen and nutrient delivery to working muscles
Improved oxidative capacity: Greater ability to use oxygen for energy production
The mitochondrion is crucial as the main organelle for energy production through the generation of adenosine triphosphate (ATP) via the electron transport system.
Cardiovascular Adaptations
Aerobic exercise leads to remarkable cardiovascular changes that increase aerobic power and improve endurance:
Enlarged cardiac dimensions: Allows for greater stroke volume
Improved contractility: Enables more efficient heart function
Increased blood volume: Permits greater filling of ventricles
Enhanced perfusion capacity: Allows for greater oxygen delivery to muscles
Arterial adaptations: Increased diameters of arteries and arterioles minimize resistance to blood flow
These adaptations collectively contribute to improved maximal cardiac output, which is crucial for endurance performance.
Training Intensity vs. Volume: What Research Shows
Recent research has begun to explore the relationship between training intensity, volume, and mitochondrial adaptations. Studies have compared different training approaches:
Long Slow-Distance (LSD): Sustained submaximal workload for long periods
High-Intensity Interval Training (HIIT): 1-4 minute all-out bouts with recovery periods
Sprint Interval Training (SIT): ~30-second maximal bouts with recovery periods
Research by Granata and colleagues found that after 4 weeks of training, SIT produced a 25% increase in maximal mitochondrial respiration, while neither LSD nor HIIT groups showed significant changes. This improvement was accompanied by changes in important regulatory proteins like PGC-1α (a key regulator of mitochondrial biogenesis), p53 (a tumor suppressor protein that also regulates mitochondrial function and cellular stress responses), and PHF20 (a transcriptional co-regulator involved in mitochondrial gene expression).
However, other studies have shown that HIIT alone can influence mitochondrial content and respiration. Jacobs et al. observed increased mitochondrial respiration and content after just 2 weeks of HIIT.
Regarding training volume, when intensity is maintained but volume is increased, different adaptations occur. Research suggests that high-intensity training is important for increasing mitochondrial activity, whereas greater training volume is needed to increase mitochondrial mass.
Protein Needs for Endurance Athletes
The protein requirements for endurance athletes have been a topic of significant research in recent years. While many athletes focus primarily on carbohydrate intake, protein plays a crucial role in recovery and adaptation.
Current Recommendations
Based on contemporary protein requirement studies:
Typical intake: 1.5 g/kg of body mass per day in male and female endurance athletes
Recommended intake: ~1.8 g/kg of body mass per day
During carbohydrate-restricted training: May need in excess of 2.0 g/kg per day
On rest days: May also require higher protein intake (>2.0 g/kg)
The literature suggests that protein requirements are higher for endurance athletes than previously thought, especially when implementing carbohydrate-restricted training strategies.
Protein Timing and Co-ingestion
The research indicates:
There's limited evidence that co-ingesting protein with carbohydrate during endurance exercise provides performance advantages
Protein co-ingestion doesn't significantly enhance glycogen resynthesis when carbohydrate recommendations are met
However, protein nutrition may play a role in optimizing adaptations during low carbohydrate/energy availability training periods
Metabolic Adaptations in Endurance Athletes
Endurance training induces substantial adaptations in metabolism, particularly related to substrate utilization and glycogen conservation.
Metabolomic Differences Between Trained and Untrained Individuals
Research has identified several metabolites that differ between endurance-trained and untrained individuals:
Acylcarnitines: C14:1 down-regulated in trained individuals
Glycerophosphocholines (GPCs): Several were differently regulated
Bile acids: Bile acids: TCDCA (taurochenodeoxycholic acid, a bile acid involved in fat metabolism and gut signaling) was down-regulated in athletes
Polyunsaturated fatty acids (PUFAs): Polyunsaturated fatty acids (PUFAs): 18S-HEPE (18-hydroxy-eicosapentaenoic acid, an anti-inflammatory lipid mediator derived from omega-3 fatty acids) showed higher levels in endurance-trained subjects.
These differences likely represent adaptations in lipid metabolism and may contribute to the lower cardiovascular risk profile observed in endurance athletes.
The Role of Epigenetics in Exercise Adaptation
Physical activity and exercise trigger adaptations in skeletal muscle through complex transcriptional responses. Epigenetics—the study of the relationship between genetics and environment—plays a crucial role in these adaptations.
Key epigenetic modifications associated with exercise include:
DNA methylation: Affects gene transcription patterns
Histone modifications: Alters chromatin structure and gene accessibility
Acetylation: Emerging as an important epigenetic modification
micro-RNA (miRNA): Regulates gene expression post-transcriptionally
These epigenetic changes facilitate adaption to exercise by modifying gene expression patterns in response to training stimuli.
Special Training Methods: HIIT and SIT
High-intensity training methods have gained popularity for their time efficiency and effectiveness:
High-Intensity Interval Training (HIIT)
Typically involves 1-4 minute all-out efforts with recovery periods
Benefits: Engages both fast-twitch and slow-twitch muscle fibers
Duration: Usually 10-30 minutes per session
Produces similar aerobic adaptations to longer traditional endurance training
Sprint Interval Training (SIT)
Consists of ~30-second maximal efforts with recovery periods
May be particularly effective for increasing mitochondrial respiration
Activates important regulatory proteins like PGC-1α and p53
These high-intensity approaches challenge the classical view of training specificity, showing that shorter, more intense workouts can effectively improve endurance capacity.
Endurance Performance and Aging
Peak endurance performance is typically maintained until about age 35, followed by modest decreases until 50-60 years, with steeper declines thereafter.
Among the physiological determinants of endurance performance (VO2 max, lactate threshold, and exercise economy), the progressive reduction in VO2 max appears to be the primary mechanism associated with age-related performance declines.
Despite these natural declines, masters endurance athletes represent remarkable examples of "exceptional aging," maintaining impressive physiological function and performance well into advanced age.
Nutrition and Supplements for Endurance Athletes
Nutrition plays a crucial role in supporting endurance performance:
Macronutrient Recommendations
Carbohydrates: Remain important for endurance athletes
Protein: Both total daily intake and post-exercise consumption are crucial for recovery and muscle maintenance
Fats: Gaining popularity, especially among ultra-endurance athletes
Hydration Guidelines
There's been a shift away from forced hydration plans toward personalized fluid intake based on thirst and sweat rates. To avoid exercise-associated hyponatremia, fluid intake should generally be limited to 300-600 mL per hour in ultra-endurance races.
Supplement Considerations
Several supplements may benefit endurance athletes:
Nitrates: May reduce oxygen cost and improve time to exhaustion
Antioxidants: Can help with recovery, especially in multi-stage events
Caffeine: Has substantial research supporting its ergogenic effects
Sex Differences in Endurance Performance
While both males and females benefit similarly from endurance training, several physiological and metabolic differences influence performance outcomes:
Cardiovascular and muscular differences: Males typically have higher VO₂ max values due to larger heart size, greater hemoglobin levels, and higher muscle mass. This contributes to greater absolute endurance performance in most sports.
Fat metabolism: Females tend to utilize a higher proportion of fat for fuel during submaximal endurance exercise, potentially preserving glycogen stores longer. This may offer an advantage in ultra-endurance events.
Hormonal influence: Estrogen has been shown to enhance lipid oxidation and mitochondrial efficiency, which may contribute to metabolic advantages in females during endurance exercise.
Fatigue resistance: Emerging research suggests that females may experience less muscle fatigue in certain endurance contexts, possibly due to differences in neuromuscular recruitment patterns and muscle fiber composition.
Despite lower absolute performance metrics on average, females often excel in ultra-endurance events and may recover faster between sessions. Training and nutrition strategies should be individualized to account for these sex-specific physiological responses.
Dangers of Excessive Endurance Training
While endurance training offers numerous health benefits, excessive training can lead to significant health risks:
Cardiac stress and remodeling: Prolonged extreme endurance exercise can cause temporary cardiac dysfunction and potentially lead to pathological heart remodeling, including atrial fibrillation and arrhythmias
Overtraining syndrome: Characterized by persistent fatigue, decreased performance, hormonal imbalances, and compromised immune function
Increased inflammation: Chronic excessive training can elevate systemic inflammation, potentially contributing to cardiovascular issues
Oxidative stress: Extreme endurance exercise generates high levels of free radicals, potentially damaging cells and tissues
Reduced bone density: Particularly in combination with caloric restriction, excessive endurance training can negatively impact bone health
Hormonal disruptions: Can cause reproductive hormone imbalances, especially in female athletes (amenorrhea)
Rhabdomyolysis risk: Extreme training can cause muscle breakdown and release of myoglobin, potentially damaging kidneys
Mental health impacts: Obsessive training patterns may contribute to exercise addiction and psychological distress
Balance in training volume and intensity, along with adequate recovery periods, is essential for maximizing benefits while minimizing these risks.
FAQs About Endurance Training
Does endurance exercise stimulate mitochondrial protein synthesis?
Yes, endurance training significantly increases mitochondrial biogenesis and protein synthesis. This adaptation is crucial for improving the body's capacity to generate energy aerobically and delay fatigue during prolonged exercise.
How does carbohydrate-restricted training affect protein needs of endurance athletes?
Carbohydrate-restricted training increases protein requirements to approximately 2.0 g/kg of body mass per day or higher. This is because when carbohydrate availability is limited, the body relies more on protein for energy and maintaining muscle mass.
Does protein nutrition improve endurance training adaptations?
Recent evidence suggests protein nutrition plays an important role in optimizing adaptations to endurance training, particularly under conditions of low carbohydrate or energy availability, which are increasingly popular periodized strategies for endurance athletes.
Does a carbohydrate-protein supplement affect endurance performance during exercise?
Current evidence doesn't strongly support performance benefits from co-ingesting protein with carbohydrate during endurance exercise, nor does it significantly enhance glycogen resynthesis when carbohydrate recommendations are already met.
How does exercise affect myofiber metabolism?
Exercise activates signaling pathways that substantially alter myofiber metabolism and skeletal muscle properties. These changes include increased mitochondrial density, enhanced oxygen utilization, and improved substrate metabolism, all contributing to better endurance capacity.
Does resistance training affect metabolomics profiles?
While this article focuses on endurance training, resistance training also influences metabolomics profiles, though with different patterns than endurance training. Resistance training tends to affect metabolites related to protein synthesis and degradation more than those involved in aerobic metabolism.
Key Takeaways
Endurance training causes significant adaptations in both cardiovascular and musculoskeletal systems, enhancing overall exercise capacity and performance.
Mitochondrial adaptations are crucial for endurance performance, with training intensity primarily affecting mitochondrial activity and training volume influencing mitochondrial mass.
Protein requirements for endurance athletes are higher than previously thought—approximately 1.8 g/kg body mass per day, increasing to over 2.0 g/kg during carbohydrate-restricted training.
High-intensity interval training (HIIT) and sprint interval training (SIT) can produce similar adaptations to traditional endurance training in less time.
Metabolomic profiles differ between endurance-trained and untrained individuals, particularly in lipid metabolism markers.
Epigenetic modifications play a key role in facilitating adaptations to endurance exercise through changes in gene expression.
Age-related declines in endurance performance are primarily related to decreasing VO2 max.
Nutritional strategies, including appropriate macronutrient intake and possibly supplements like nitrates and caffeine, can support endurance performance.
Call to Action
Ready to optimize your endurance training? Here's how to put this research into practice:
Incorporate varied training intensities: Include both high-intensity intervals and longer, lower-intensity sessions to maximize mitochondrial adaptations.
Review your protein intake: Ensure you're consuming enough protein (1.8+ g/kg/day) to support recovery and adaptation, especially if implementing carbohydrate restriction.
Consider your training phase: Periodize your approach to include both high-intensity work for mitochondrial activity and sufficient volume for mitochondrial mass.
Personalize your nutrition: Develop an individualized nutrition plan that supports your specific training demands, considering appropriate supplements if needed.
Monitor adaptations: Track your performance metrics over time to ensure your training is producing the desired physiological adaptations.
By implementing these science-based strategies, you can enhance your endurance performance regardless of your current fitness level or age. Remember that the most effective training plan is one that you can consistently follow, so find approaches that work for your lifestyle and goals
Related Articles
Citations
Witard, O.C., Hearris, M. & Morgan, P.T. Protein Nutrition for Endurance Athletes: A Metabolic Focus on Promoting Recovery and Training Adaptation. Sports Med (2025). https://doi.org/10.1007/s40279-025-02203-8
Mallett G. (2025). The effect of exercise and physical activity on skeletal muscle epigenetics and metabolic adaptations. European journal of applied physiology, 125(3), 611–627. https://doi.org/10.1007/s00421-025-05704-6
Working out the chemistry of exercise endurance | NIH Intramural Research Program. (2025, March 17). https://irp.nih.gov/blog/post/2025/03/working-out-the-chemistry-of-exercise-endurance
Bottura, R. M., & Dentillo, D. B. (2025). Genomics May Be the Key to Understanding Endurance Training Pillars. Genes, 16(3), 338. https://doi.org/10.3390/genes16030338
Weiss, A., Alack, K., Klatt, S., Zukunft, S., Schermuly, R., Frech, T., Mooren, F. C., & Krüger, K. (2022). Sustained Endurance Training Leads to Metabolomic Adaptation. Metabolites, 12(7), 658. https://doi.org/10.3390/metabo12070658
Goh F. Q. (2021). Can too much exercise be dangerous: what can we learn from the athlete's heart?. The British journal of cardiology, 28(3), 30. https://doi.org/10.5837/bjc.2021.030
Hughes, D. C., Ellefsen, S., & Baar, K. (2018). Adaptations to Endurance and Strength Training. Cold Spring Harbor perspectives in medicine, 8(6), a029769. https://doi.org/10.1101/cshperspect.a029769
Santisteban, K. J., Lovering, A. T., Halliwill, J. R., & Minson, C. T. (2022). Sex Differences in VO2max and the Impact on Endurance-Exercise Performance. International journal of environmental research and public health, 19(9), 4946. https://doi.org/10.3390/ijerph19094946
https://www.physio-pedia.com/Endurance_Exercise
Disclaimer
The information on this website is for informational purposes only and is not intended to be a substitute for professional medical advice, diagnosis, or treatment. Always seek the advice of your physician or other qualified health care provider with any questions you may have regarding a medical condition or treatment. Never disregard professional medical advice or delay in seeking it because of something you have read on this website.
About the Author:
Dr.T.S. Didwal, MD, is an experienced Internal Medicine Physician with over 30 years of practice. Specializing in internal medicine, he is dedicated to promoting wellness, preventive health, and fitness as core components of patient care. Dr. Didwal’s approach emphasizes the importance of proactive health management, encouraging patients to adopt healthy lifestyles, focus on fitness, and prioritize preventive measures. His expertise includes early detection and treatment of diseases, with a particular focus on preventing chronic conditions before they develop. Through personalized care, he helps patients understand the importance of regular health screenings, proper nutrition, exercise, and stress management in maintaining overall well-being.